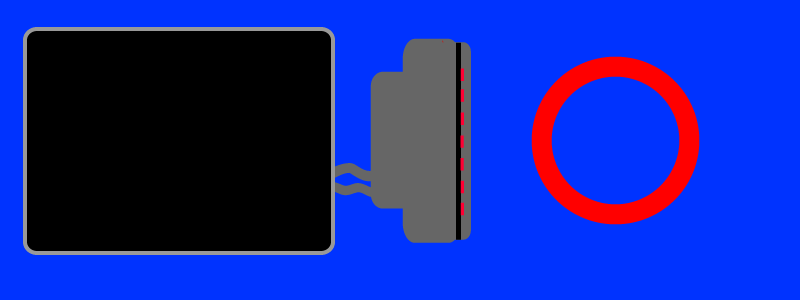
We will discuss the following aspects. Please scroll down and start reading.
- Introduction to sound and ultrasound
- Sending and receiving ultrasound
- Interaction of ultrasound with body tissues
- Scanning modes : A scan
- Scanning modes : B scan
- Frequency, Wavelength, Resolution, and Depth
- Doppler Effect
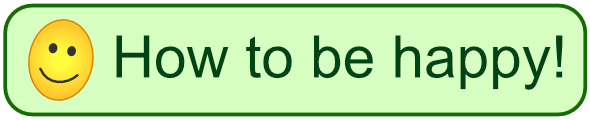
Happiness is often not easy to achieve in our modern stressful world. The free website at the link below explains in an easy to understand way, ancient and modern ideas about how to be happy. All the ideas are very practical so that you can use them in your day to day life. You are welcome to visit the website and benefit from it. It is completely free.
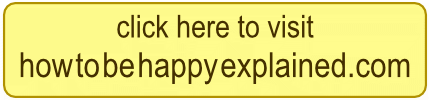
Sound and Ultrasound
We are all familiar with sound. It helps us to communicate and listen to gossip. Audible alarms warn us and pulse oximeters sing saturations to us.
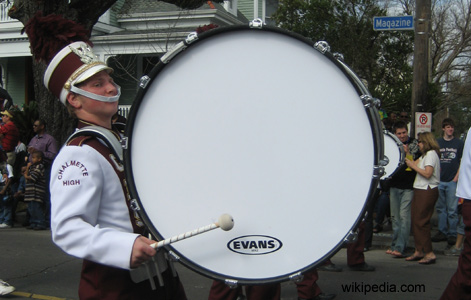
But most amazing is that sound can actually help us to see what is hidden, just like the way bats ‘see’.
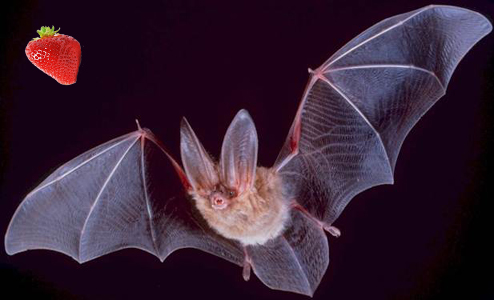
Unfortunately , bats always have the night shift. They go hunting for things to eat at night where food isn’t well lit. A juicy red strawberry would not easy to spot at night.
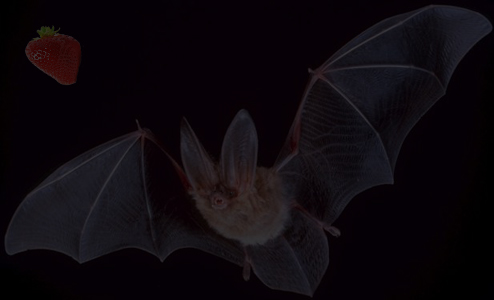
Fortunately, bats are gifted with a system of locating things with sound. First they emit sound.
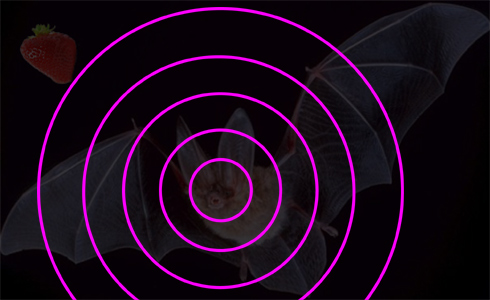
The sound waves bounce on objects such as the strawberry. The sound waves that bounce on the strawberry reflect back to the bat. The direction from where the sound returns tells it in which direction the strawberry is. The time taken for the sound waves to reach the strawberry and return back to the bats ear tells it how far the strawberry is. The longer the sound waves take to return, the further away is the strawberry.
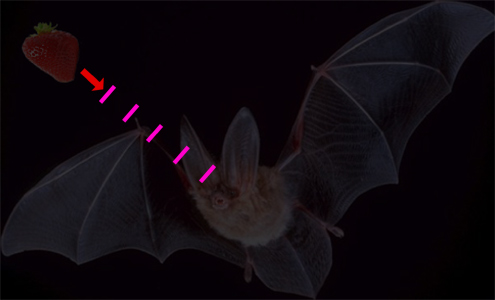
So using sound, the bat can find the unseen. In a similar way, we in anaesthesia can also use sound to locate the unseen. For an example, we can use it to guide our needle when inserting a central venous catheter into the internal jugular vein in the neck of a patient without damaging other vital structures.
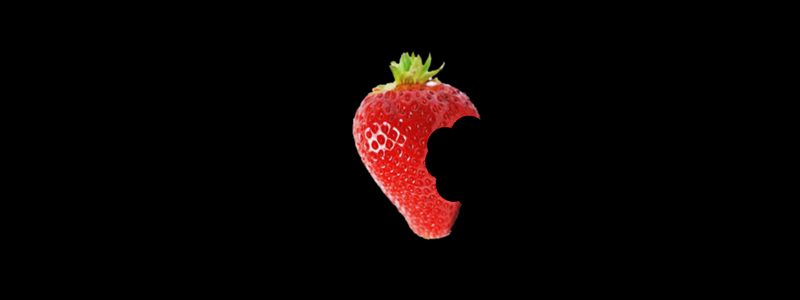
What is “sound” and “ultrasound “?
Sound is a vibration that is transmitted in a medium (e.g. air), that can be heard by an human ear. If you were an ant or a bird, the meaning of sound would be different. Sound specifically refers to what the human ear can hear.
All vibrations, including sound, have a frequency. Frequency is a measure of how often something “vibrates “ per second. The unit of frequency is Hertz, the official symbol being Hz, and can be thought of as “vibrations per second “ (this not the official definition!). The human ear can hear between frequencies of about 20 Hz to 20,000 Hz. So “sound “ is vibrations in this frequency range.
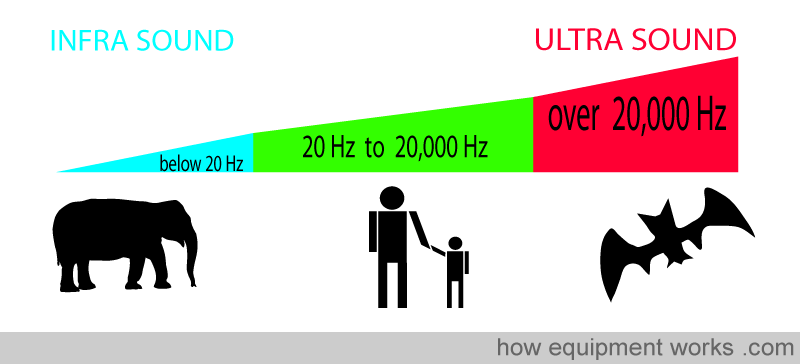
The human ear cannot hear below 20 Hz and frequencies below this are called “infra sound “. Elephants can use infra sound and use it to communicate jokes that they don’t want humans to hear.
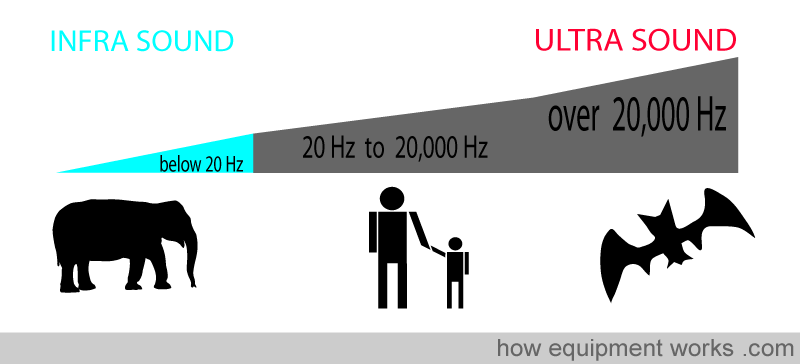
The human ear cannot hear above 20,000 Hz. Frequencies above 20,000 Hz are called “ultrasound “. Bats use ultrasound to locate food as described above and dolphins use it to communicate with their friends. And importantly, ultrasound is used in anesthesia for imaging various parts of the body. Ultrasound used in medical imaging typically operate at frequencies way above human hearing: about 2 million Hz to 20 million Hz (2-20 MHz).
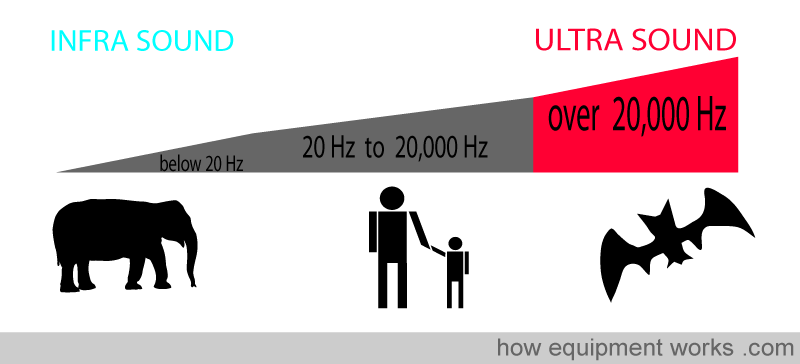
Generation of Ultrasound Waves
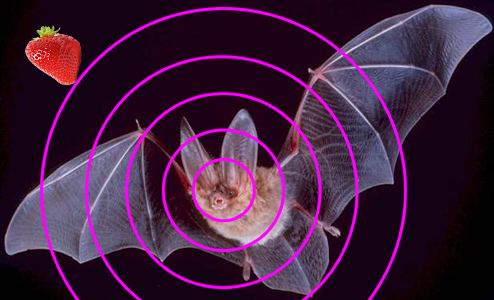
To use ultrasound to find things, we first need to have a way of generating them. We need something to create vibrations that will travel in the tissues in a patient.
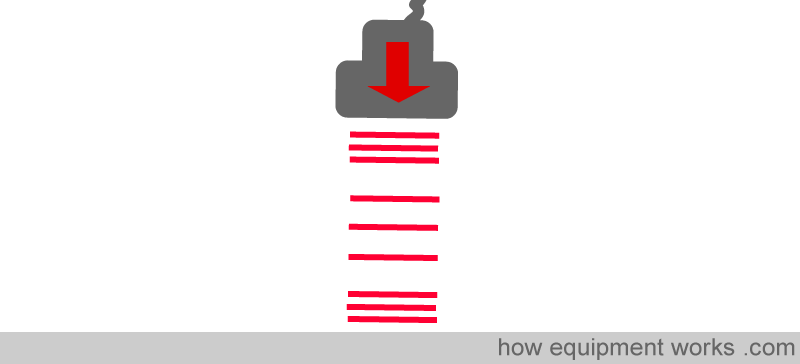
There is a special material called a “piezo electric crystal”. This material has a very special property. When a voltage is applied to an piezo electric crystal (shown in red below), it expands. When the voltage is removed, it contracts back into its original thickness.
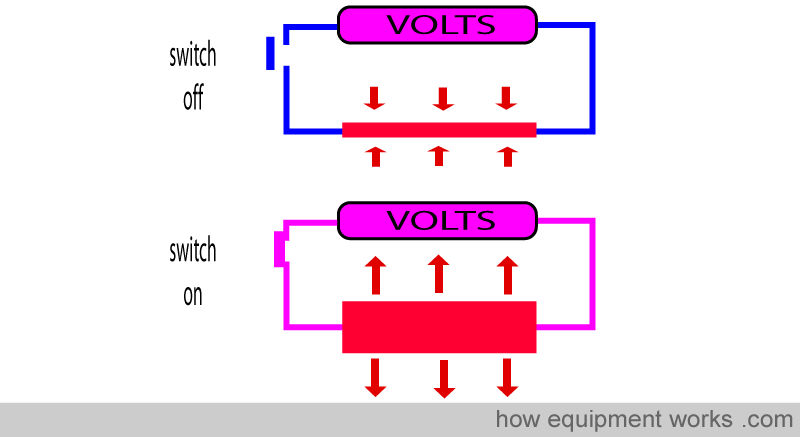
If the voltage is rapidly applied and removed repeatedly, the piezo electric crystal rapidly expands and relaxes, creating ultrasound waves.
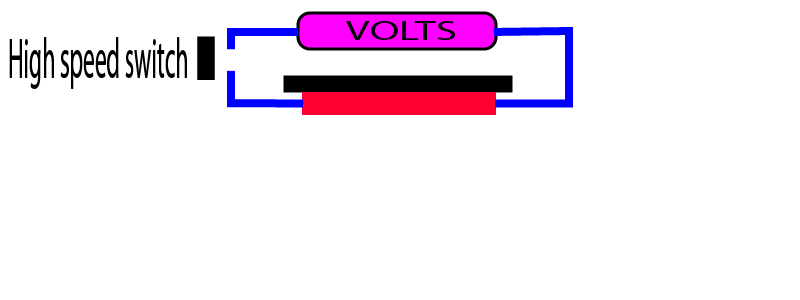
Receiving Ultrasound
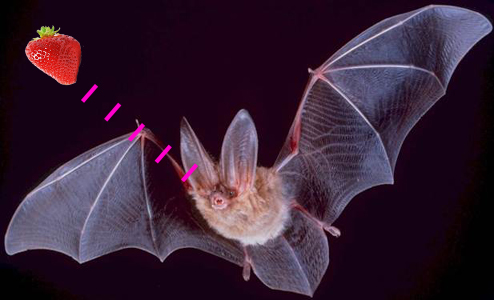
To locate something using ultrasound, one needs to have a way of listening to the sound waves that are bounced off various objects. In the previous section, we discussed how a piezo electric crystal expands when a voltage is applied to it and how that is used to generate ultrasound waves. In addition to this, piezo electric crystals have another very useful property that enables it to be also used for receiving ultrasound waves. When a piezo electric crystal is compressed, it generates a voltage. This property is used to “listen “ for the ultrasound waves that return after striking objects. When returning sound waves hit the piezo electric crystal, it gets compressed. The crystal then generates a voltage that corresponds to the intensity of the ultrasound wave that hits it.
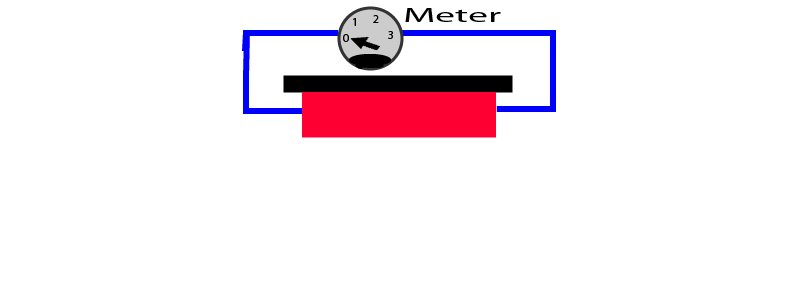
The ultrasound machine conveniently uses the same piezo electric crystal to transmit and to listen to ultrasound waves. First the machine applies a voltage to the crystal to expand it and transmit.
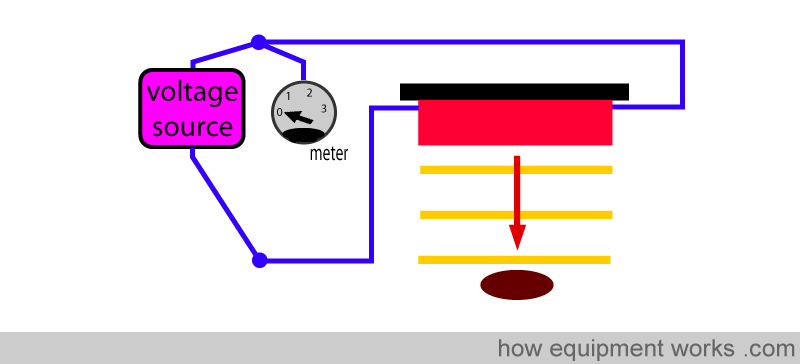
The ultrasound machine then very quickly switches to a listening mode by monitoring the voltage across the piezo electric crystal. This transmit and receive cycle is repeated very rapidly.
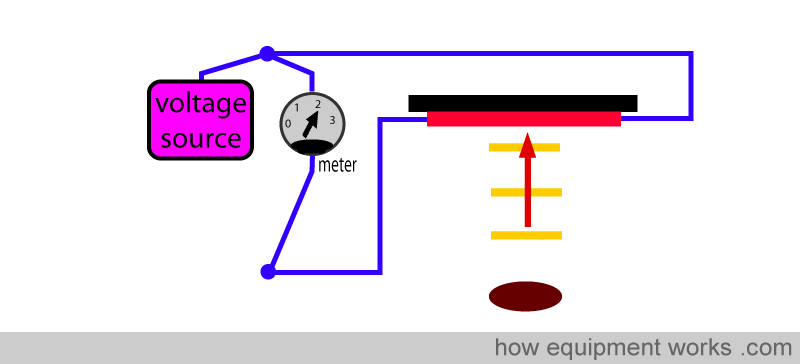
The above examples show only one crystal for clarity. In reality, ultrasound probes are composed of a large number of individual piezo electric crystals. The information gathered from the crystals are processed by a computer to display the images on a screen.
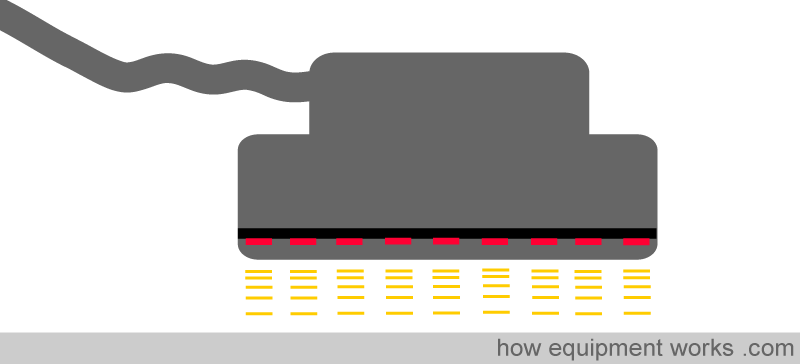
Jelly
Air is the enemy of ultrasound. Ultrasound waves tend to reflect strongly wherever air meets biological tissue. If there is even a small bubble between the probe and the patients skin, the ultrasound waves will be reflected away instead of penetrating the skin. Without the waves going into the patient, you will not be able to get a descent image. Therefore, it is absolutely vital to make sure that there are no air bubbles between the probe and the skin of the patient.
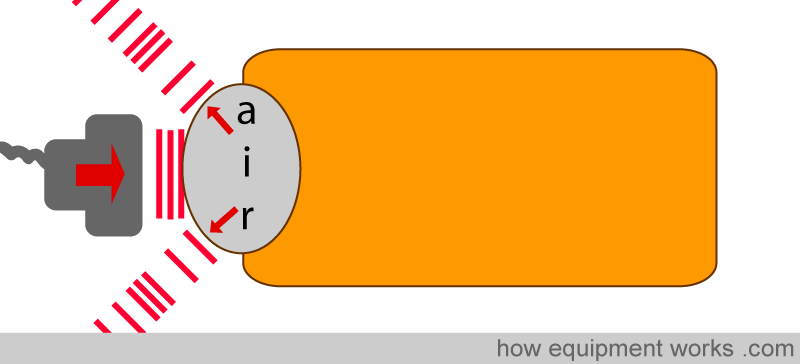
On the other hand, ultrasound travels very easily through liquids. For this reason, it is common to use a thick liquid ( jelly ) between the probe and the patients skin. The thick liquid helps to keep away air bubbles and allows easy passage of the ultrasound waves.
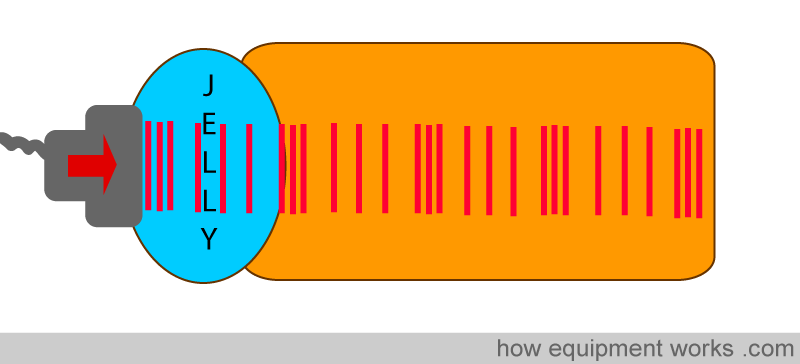
What happens to the ultrasound waves ?
Once the ultrasound enters the body, different things can happen to them. Only some of it returns back to the probe to help the machine form a image. The rest is lost. When ultrasound enters the body, some of it undergoes:
1. attenuation
2. refraction
3. reflection
Attenuation
Some of the ultrasound waves are attenuated. That is, the body absorbs the ultrasound energy, making the waves disappear. These waves don’t return to the probe and are therefore “wasted”. The more the body tissues that the ultrasound waves have to cross, the more attenuation the waves suffer. That is one reason why it is more difficult to image deeper structures.
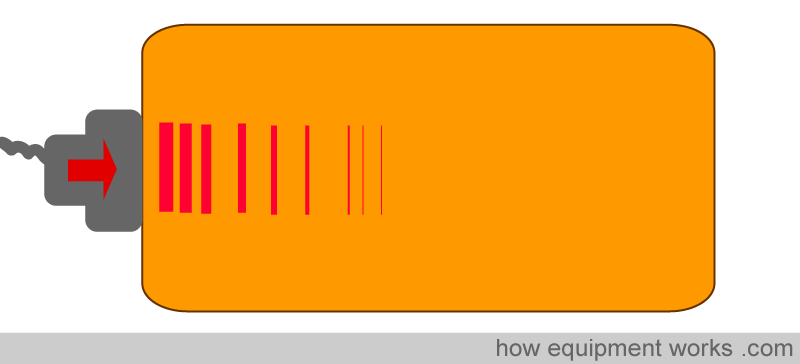
Refraction
Every substance , such as a nerves, muscles, or fat, has a unique property called “acoustic impedance”. Acoustic impedance is a somewhat complicated concept, but basically depends on the density of the substance and the speed of ultrasound in that substance. Substances with different acoustic impedances alter the course of ultrasound waves in an important manner.
When an ultrasound wave (shown as a red arrow in image below) tries to pass from one substance to another substance with a different acoustic impedance, two things happen to it.
Part of the ultrasound waves continues into the second substance, but becomes slightly bent away from their original direction (pink arrow). The bending away when ultrasound passes from one substance to another substance with a different acoustic impedance is called refraction.
In addition to this, another very important thing happens. Part of the wave (shown as blue arrow in image below) is reflected back to the probe .The amount reflected back depends on the difference of the acoustic impedance between the two substances, more the difference, more the reflection. Reflected waves are extremely important, since it is only these waves that return back to the probe and provide information for the machine to show an image.
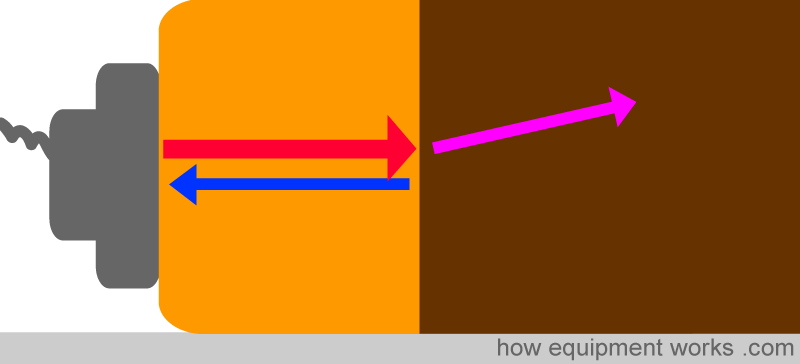
As the ultrasound wave crosses from one tissue to the next, each with a different acoustic impedance, some of the wave is reflected back at each crossing (two blue arrows in image below). Therefore, multiple reflected waves return to the probe and the machine uses this information to display an image showing the different tissues.
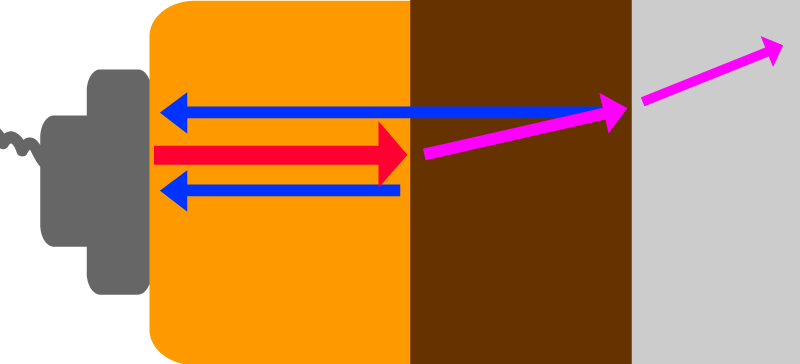
Reflection
Irregular surfaced objects such as nerves scatter the ultrasound waves in all directions. A small portion of the waves are reflected back to the probe (shown as blue arrow in image below). This is called “scattered reflection”.
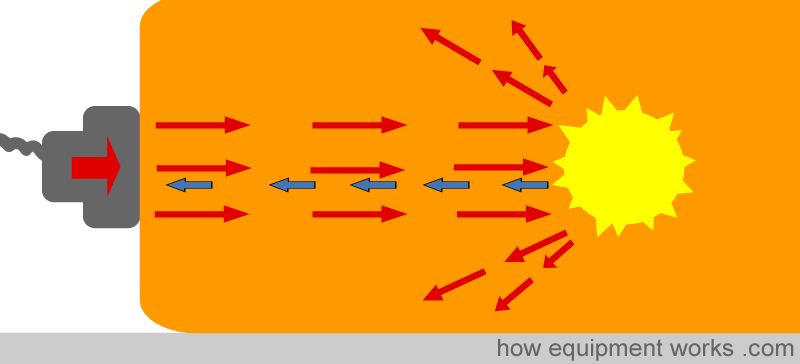
If an object is large and smooth like a nerve blocking needle, all the ultrasound wave is reflected back. This is very useful since it helps us to clearly see needles when performing ultrasound guided nerve blocks. This mirror like reflection, where the waves are reflected back mostly in one direction is officially called “specular reflection”.
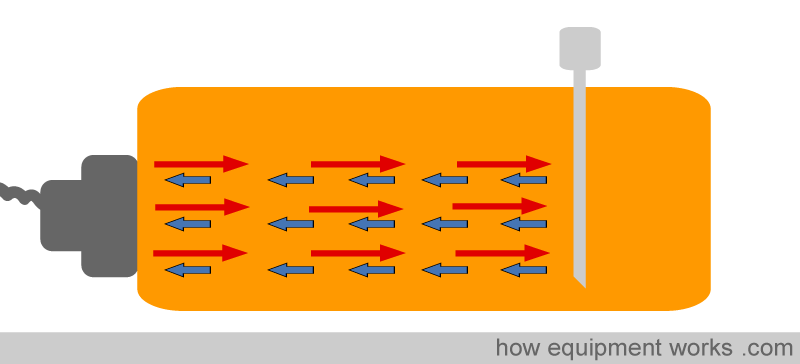
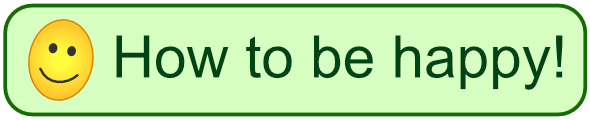
Happiness is often not easy to achieve in our modern stressful world. The free website at the link below explains in an easy to understand way, ancient and modern ideas about how to be happy. All the ideas are very practical so that you can use them in your day to day life. You are welcome to visit the website and benefit from it. It is completely free.
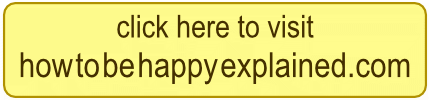
Ultrasound Scanning Modes
When we look at things with our eyes, there are various ways in which we “look “. At times, we might choose to look only straight ahead like when we read a notice on a wall.
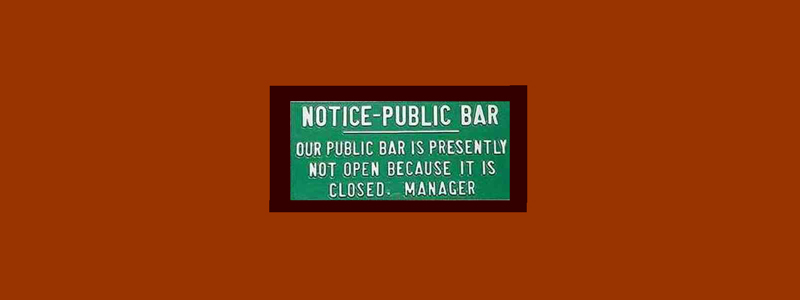
Or we might look horizontally when scanning the sea.
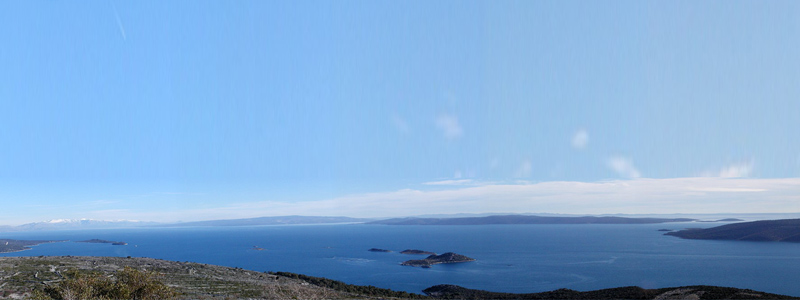
Or we might scan the whole area, up and down, left and right, in many dimensions when absorbing scenery such as the one below in Sri Lanka.
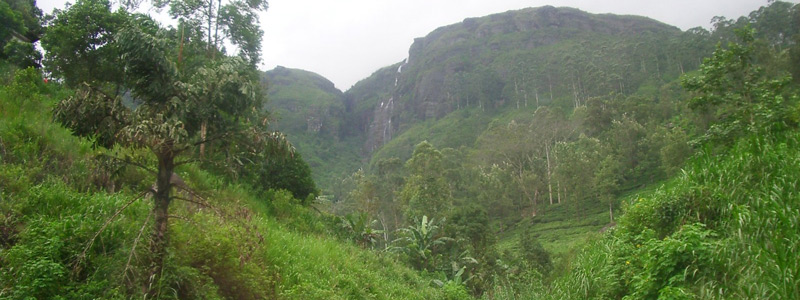
In a similar way, there are many different ways a ultrasound probe can “look “ at things. These ways are called “modes “ and these will be described below. The modes are named with letters and may sound very confusing. However, we will discuss each in turn and you will, at the end, understand the basics of them.
A mode (Amplitude mode)
B mode (Brightness mode) including real time, 2 dimensional, B mode
M mode (Motion mode)
A Mode Scanning
The A mode is the simplest form of ultrasound imaging and is not frequently used. The ultrasound wave that comes out of the probe travels in a narrow pencil like straight path.
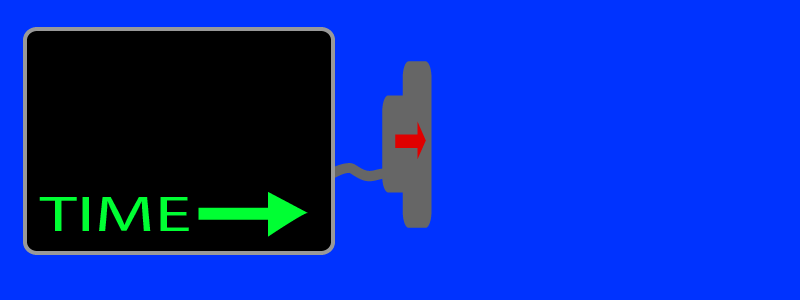
One use of the A scan is to measure length. For an example, ophthalmologists can use it to measure the diameter of the eye ball. Imagine that the red circle below is the eye ball and you want to measure the diameter of it.
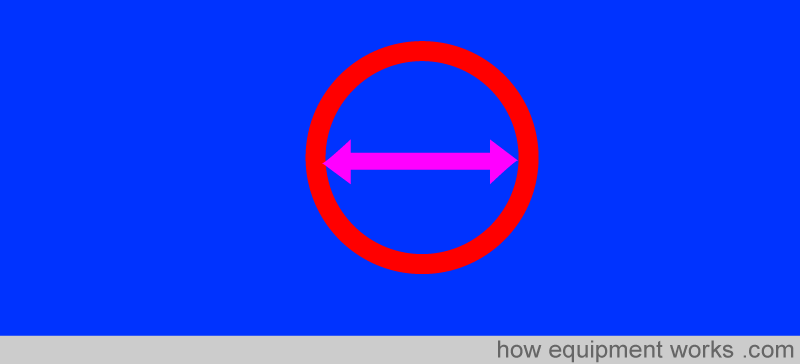
An ultrasound machine scanning in “A scan” mode can be used. The probe is placed on one end of the eye ball.
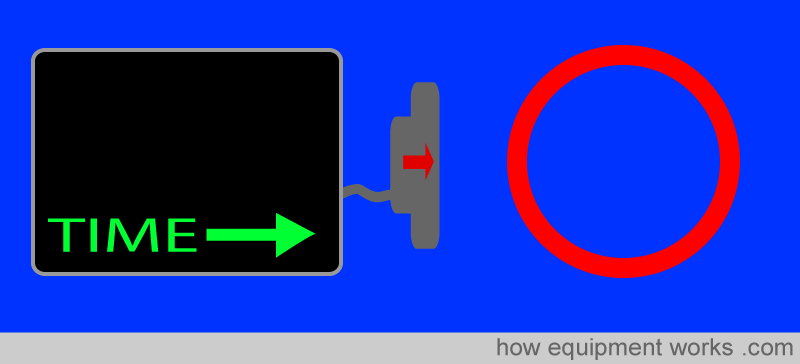
An ultrasound wave is sent from the probe and at the same instance, a line from the left of the screen starts to be drawn. This line moves horizontally measuring time.
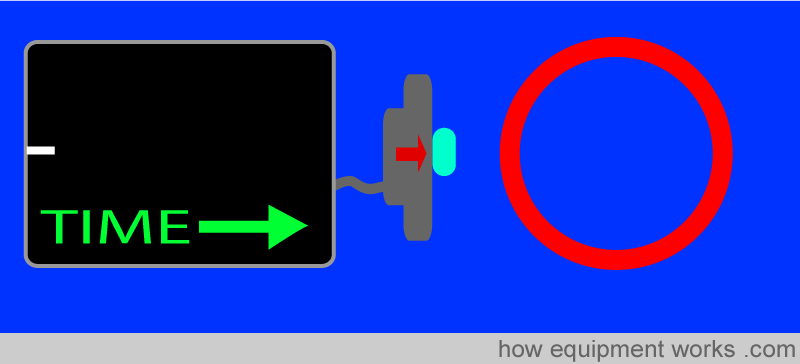
As the wave reaches the first wall of the eye, some of the ultrasound is reflected back into the probe. The returned wave is recorded on the line as a bump. The stronger is the returned wave, higher the height of the bump. The height of the bump is called Amplitude which is what the “A” of “A scan” stands for.
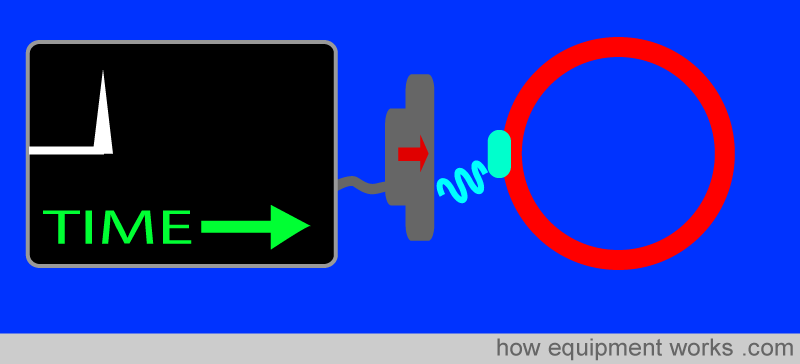
The ultrasound wave continues further in the eye.
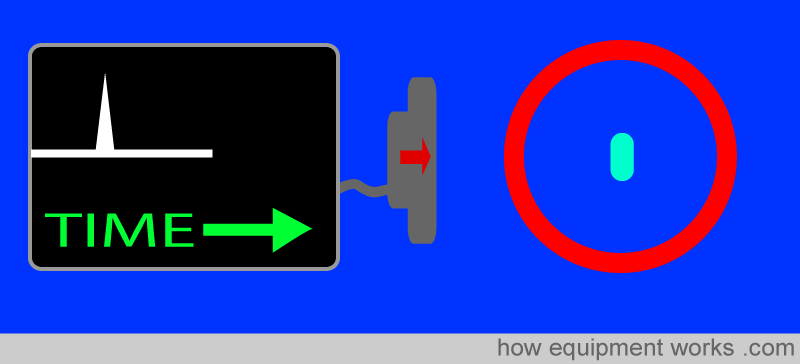
The wave then meets the wall that is furthest away. Again some of the ultrasound wave is reflected back into the probe and another bump is drawn.
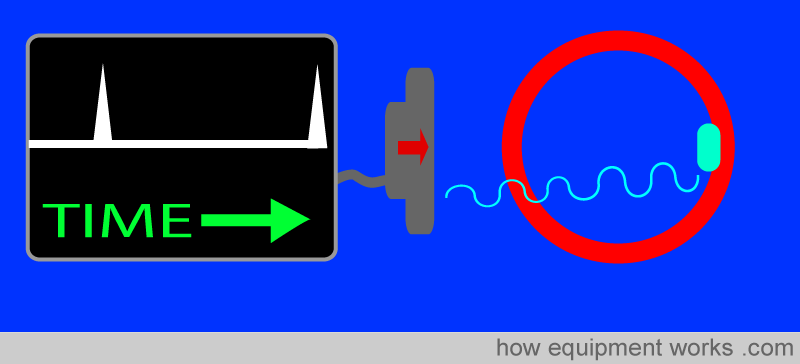
The time difference between the first bump and the second bump represents how long the ultrasound wave took to travel between the two walls. Longer the length, longer is the time difference. The speed of ultrasound in the eye is known to be 1500 meters per second (yes, that is fast). So if you know the time difference (given by the interval between the two bumps), you can calculate how far the wave traveled between the two walls of the eye, giving you the eyeball length.
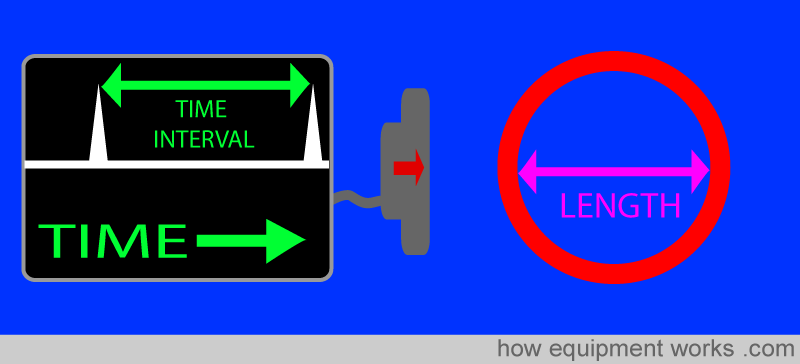
B Mode Scanning
In its simplest form, the B scan mode is very similar to the A scan mode. Just like the A scan, a wave of ultrasound is sent out in a pencil like narrow path. And again like the A scan, the horizontal line represents the time since the wave was released.
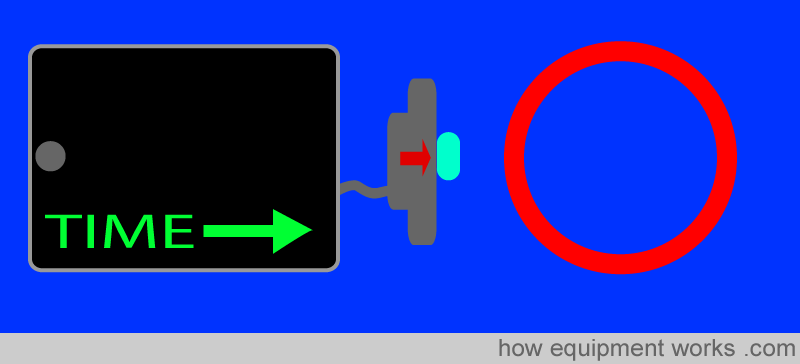
Again using the eye ball as an example, the probe is placed on one end. Like in the A scan, when the wave meets the first wall, a part of the wave is reflected back into the probe. However, this time, instead of a bump, the strength of the returning wave is recorded by a bright dot. The brightness of the dot represents the strength of the returning wave. The brighter the dot, the stronger is the returning wave. The letter “B” of “B scan” represents Brightness.
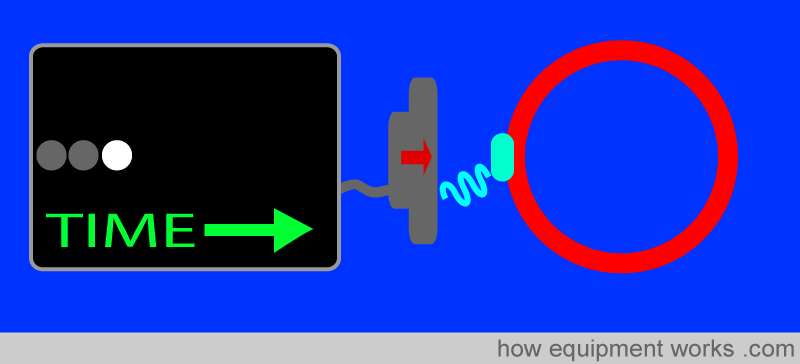
The wave continues.
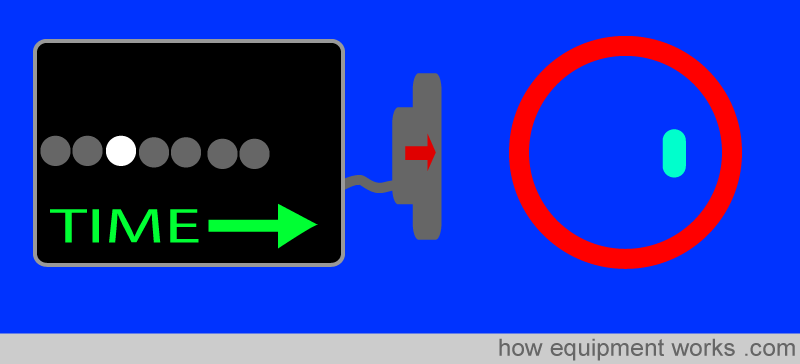
And when the wave reaches the other wall, again part of it is reflected back into the probe. This returning wave, like the returning wave from the previous wall, is represented as a bright dot on the screen.
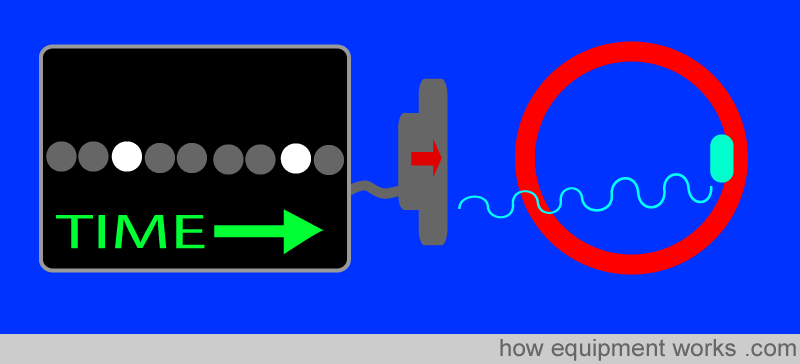
The B scan in the form discussed doesn’t amount to much …. just a few dots of different brightness along a line. However, if a B scan is done at different levels of the object, you will get a two dimensional image on the screen as shown below. First a B scan is done at the top of the structure chosen, e.g. the eye.
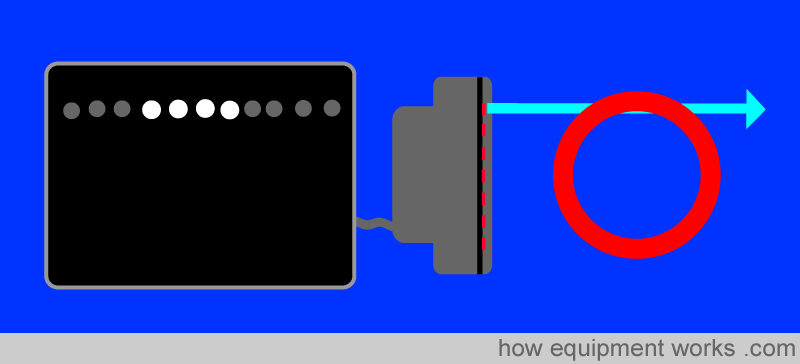
The first B scan line is kept on the screen. Then at a slightly different level, the B scan is repeated.
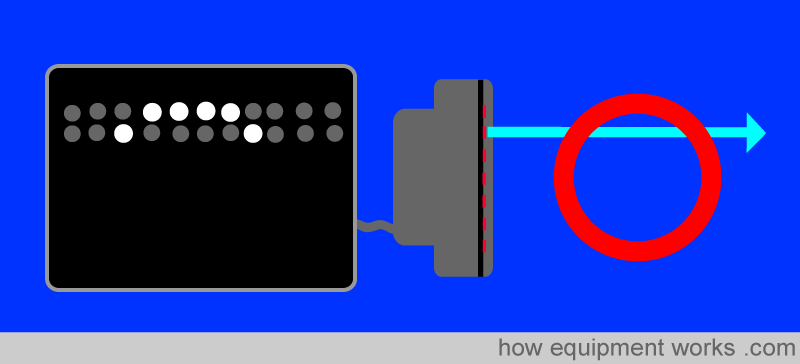
This B scan result is also kept on the screen.Then, again at a slightly different level, the B scan is repeated.
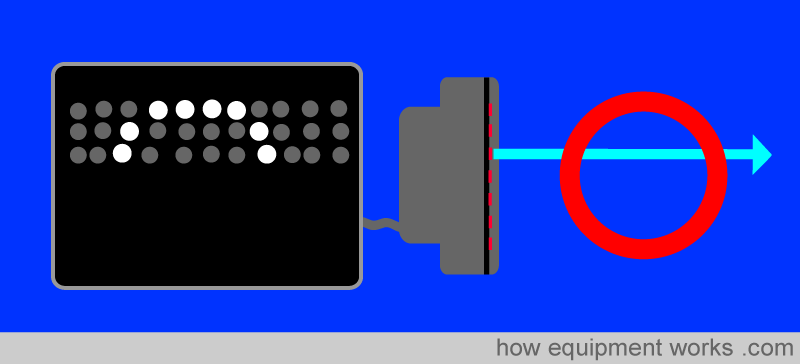
In this way, a two dimensional (2 D) image of the object is formed on the screen.
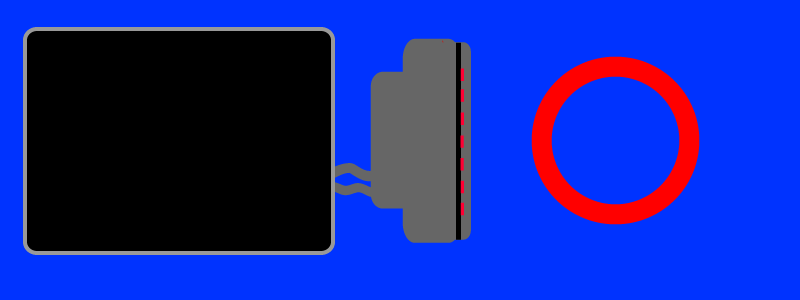
In real life, the process happens very quickly. The structures are scanned and the image redrawn many times a second.
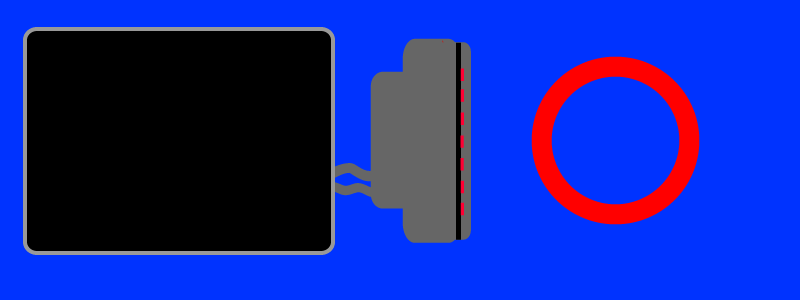
Since the image is redrawn so rapidly, one can see size changes (e.g. pulsations of carotid artery) in “real time” (i.e. as it happens) .The B scan is the commonest mode of ultrasound that we see in anesthesia. The complete description of the mode is “real time , 2 dimensional (2 D), B scan”. The 2 dimensional (2D) refers to the fact that the image has two dimensions; horizontal (X axis) and vertical ( Y axis).
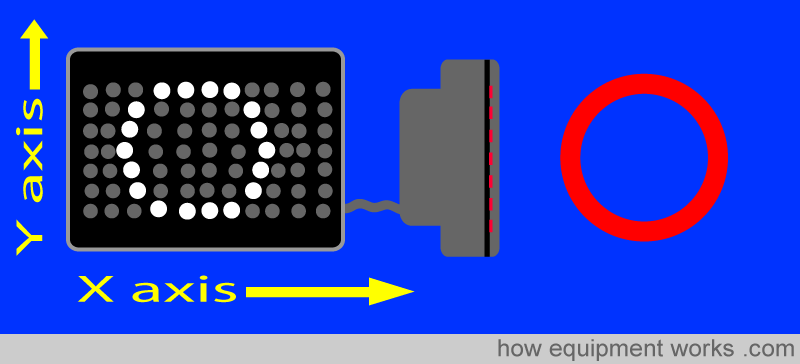
M Mode Scanning
This mode is mostly used in cardiology and will not be described.
Frequency, Wavelength, Resolution, and Depth
Frequency, wavelength, resolution, and depth are interlinked. These seem to be complex physics stuff to grasp, but it is worth understanding them because it can help you to get the best images from your ultrasound machine.This website will simply it all for you so don’t worry.
The first step is to understand what the terms mean. Each of the terms will be described to you, starting with “frequency”.
Frequency
As you may recall, ultrasound waves are created by a vibrating piezo electric crystal. The vibration creates alternate high pressure and low pressure areas which travel forwards.
Frequency is the number of high pressure / low pressure cycles in a period of one second.
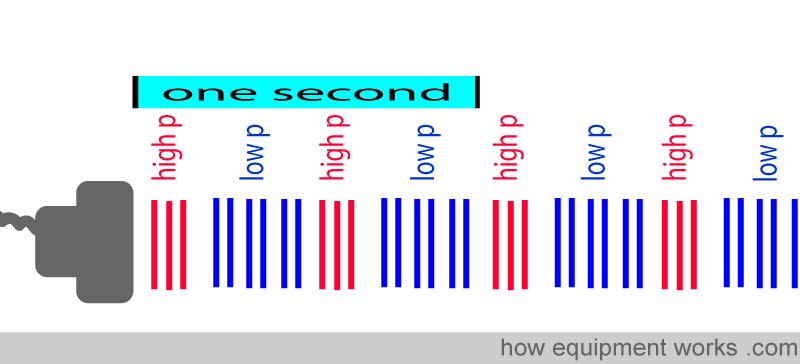
In the image below, the high frequency wave has an higher number of cycles of high /low pressure areas in a period of one second. Similarly, the low frequency wave has a lower number of cycles of high / low areas of pressure per second.
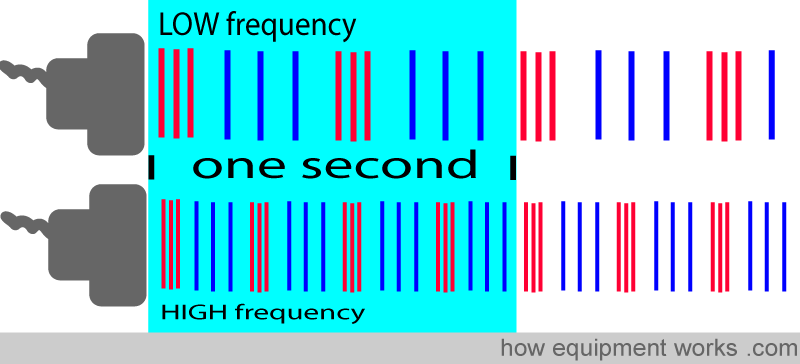
The unit of frequency is Hertz, which is abbreviated to Hz. Hz refers to the “number of cycles per second”. In the image below, the low frequency has 2 high /low pressure cycles per second. In other words, the low frequency wave below has a frequency of 2 Hz. Similarly, the high frequency wave below has 4 high /low pressure cycles per second. In other words, the high frequency wave below has a frequency of 4 Hz. These small numbers have been used only to explain the concept of frequency to you. In reality, ultrasound operates at many million cycles per second. (e.g. about 2 million Hz to 20 million Hz (2-20 MHz)
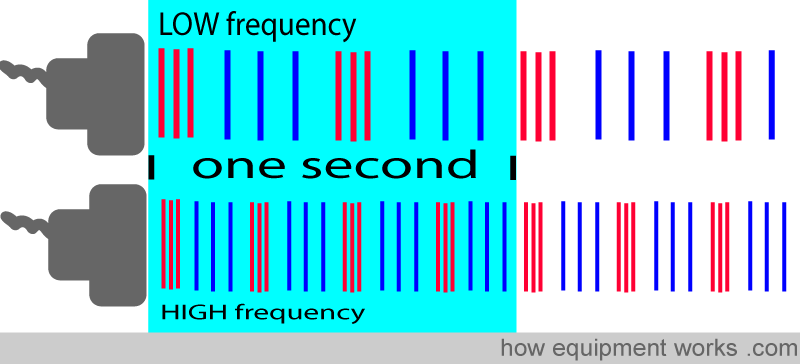
Wavelength
Wavelength is the distance between identical points in adjacent cycles of a waveform. For an example, you can measure wavelength by measuring the distance between two adjacent highest pressure points in the wave.
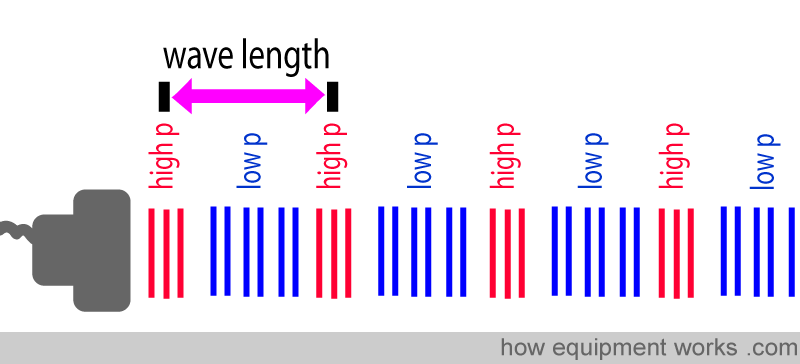
Or similarly, you could have measured the distance between two adjacent lowest pressure points to get the wavelength.
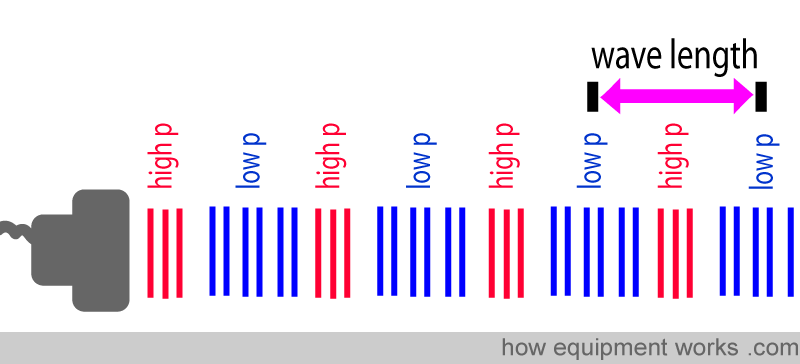
In either case, the wavelength is the same for a given wave.
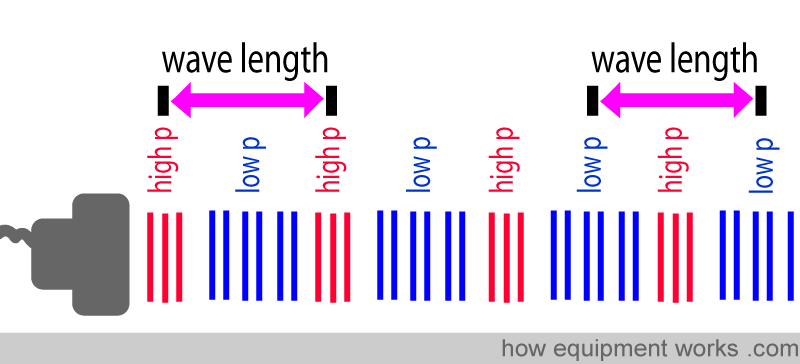
Relationship between Wavelength & Frequency
Wavelength and frequency are importantly interrelated. When the frequency is increased the wavelength becomes shorter. Similarly, when the frequency is decreased, the wavelength becomes longer. This has some important implications when you use ultrasound in clinical practice and this will be explained to you later.
First let us discover why high frequency waves have a shorter wavelength. In the following image, the upper wave has a higher frequency. You will recall that high frequency means higher cycles per second (one cycle = one high pressure area followed by one low pressure area). In this example, the high frequency wave has four cycles per second (the high pressure areas are shown with a purple dot). Similarly, the lower wave has a lower frequency and therefore has less cycles per second.
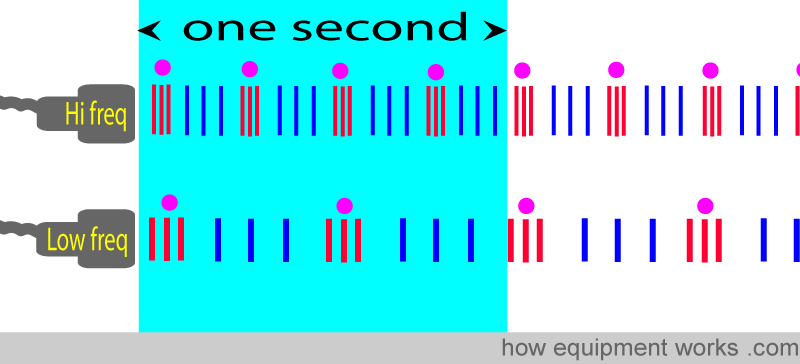
This means that the high frequency wave has more cycles that are “squeezed” into the one second time frame. The low frequency wave has less cycles “squeezed” into the same one second time frame. Thus the cycles in a low frequency wave are more more wide apart. As discussed before, wavelength can be measured between two adjacent high pressure areas of a wave. You can now see that low frequency waves have a longer wavelength. High frequency waves have a shorter wavelength.
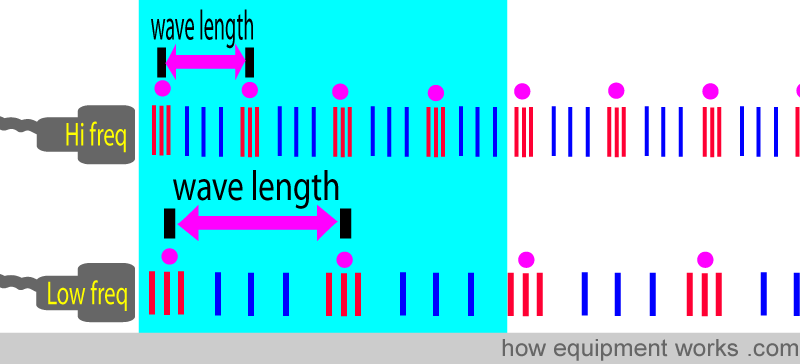
In more “serious” physics terms, one would describe this as “wavelength is inversely proportional to frequency”. This can be expressed as the equation shown in the yellow square below:
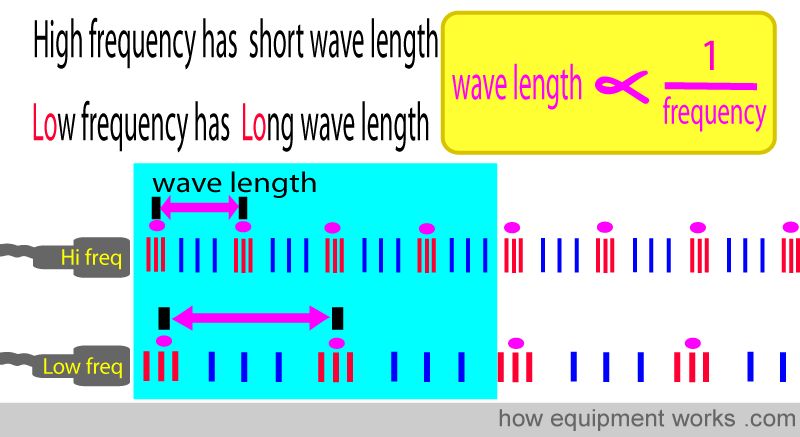
Resolution
We have discussed frequency and wavelength. These can affect “resolution”. So let us understand what resolution means.
Resolution is the ability to see two things as two things.
If the resolution is good, the picture will be clear and the two objects will look like two objects.
If the resolution is poor, the picture will be blurred and the two objects will look like one.
Our aim is to get the best possible resolution from our ultrasound machine. Higher the resolution, higher will be the quality of the image we see.
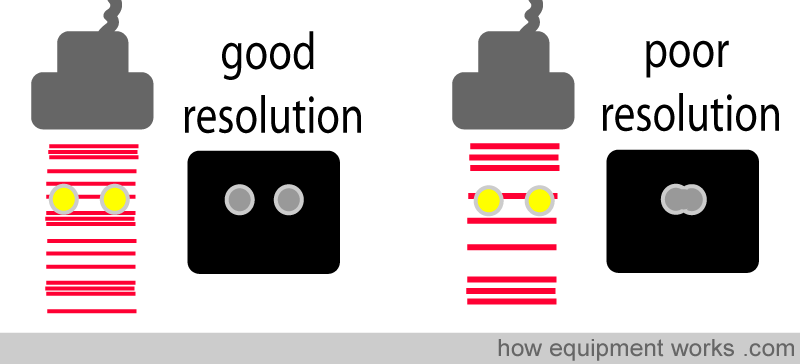
For slightly complex physics reasons, shorter wavelengths give a better resolution.
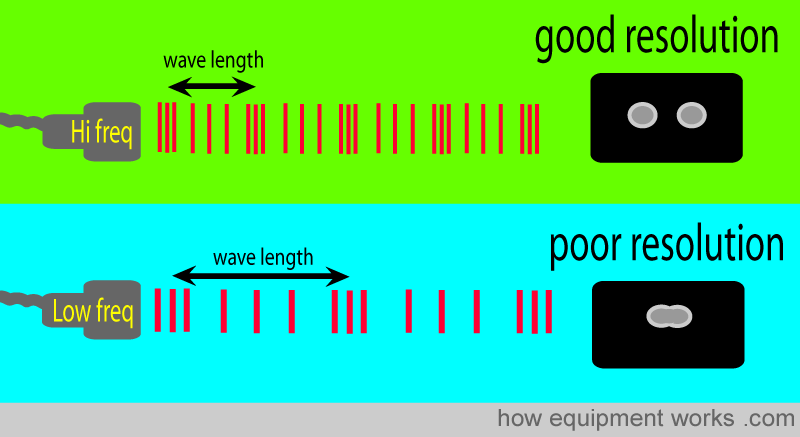
But we also know that wavelength and frequency are related to each other. To get a shorter wavelength we should use a high frequency.
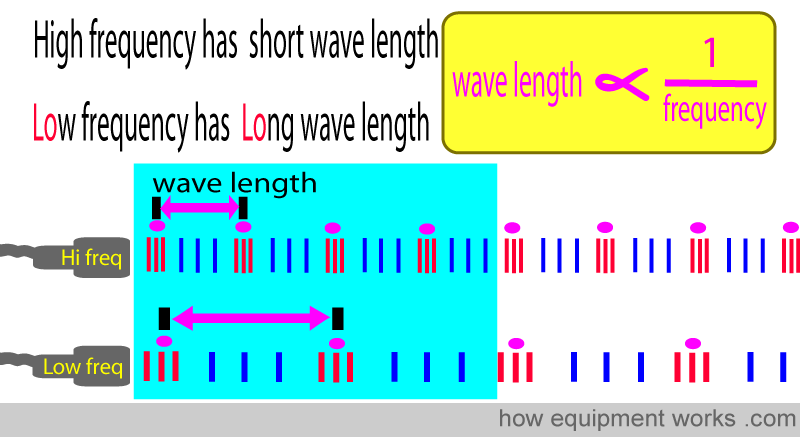
So to get a good resolution, we should use a high frequency because a high frequency has a short wavelength.
i.e. high frequency = short wavelength = good resolution
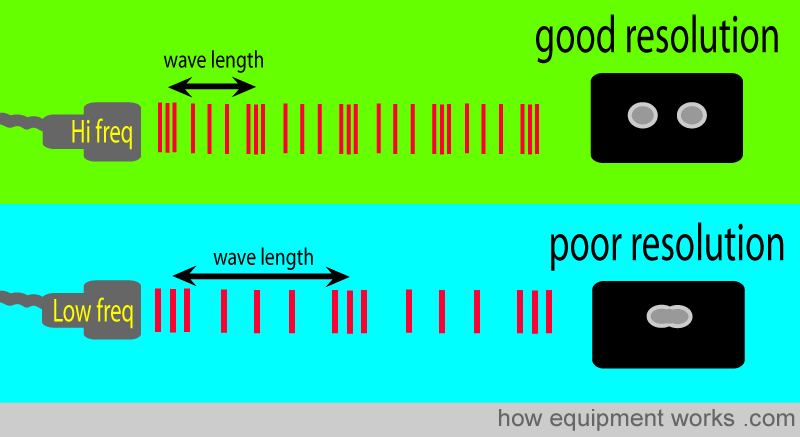
Depth
If the ultrasound waves do not penetrate the body to a sufficient depth, you may miss seeing the structure that you want to see.
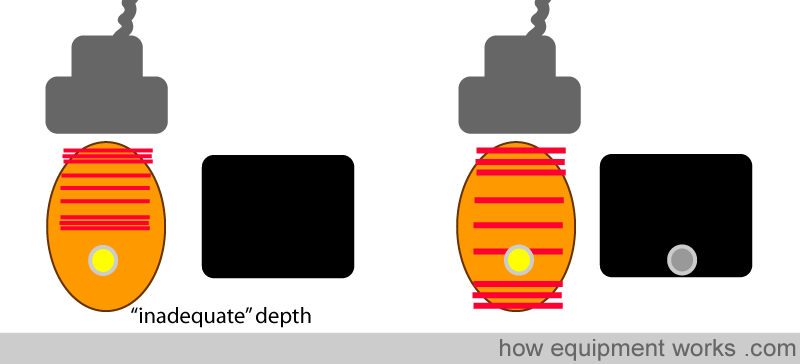
The depth of penetration is related to the frequency of the ultrasound wave. Higher frequencies have a shorter depth of penetration. Lower frequencies have a longer depth of penetration.
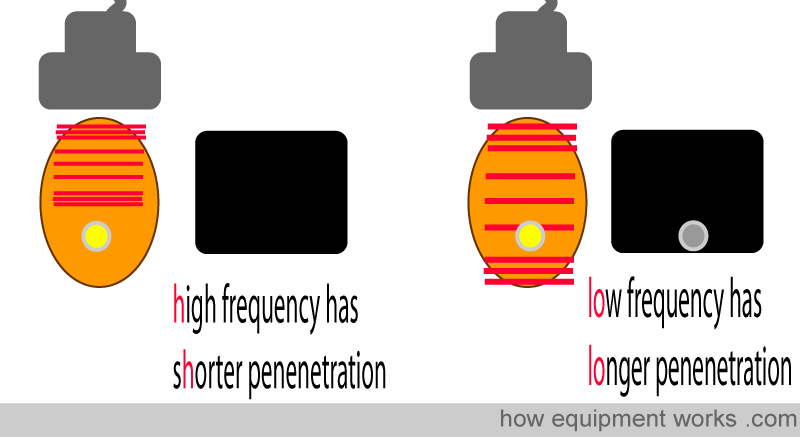
So in clinical practice, you might think that using the lowest frequency is the best because it gives a longer depth of penetration. However it isn’t as straightforward as the next section will explain.
Finally linking it all : Frequency, Wavelength, Resolution, and Depth
Now that you have persisted in understanding frequency, wavelength, resolution and depth, let us try and combine them in a meaningful way.
For ultrasound to be clinically useful, we want sharp pictures. I.e. we want the best resolution.
Additionally, we want adequate depth of penetration to see deeper structures.
The physics of ultrasound is such that both these requirements cannot be fully met at the same time. For a good resolution, we need a short wavelength, which means we need a higher frequency. Unfortunately, an higher frequency also means a shorter penetration.
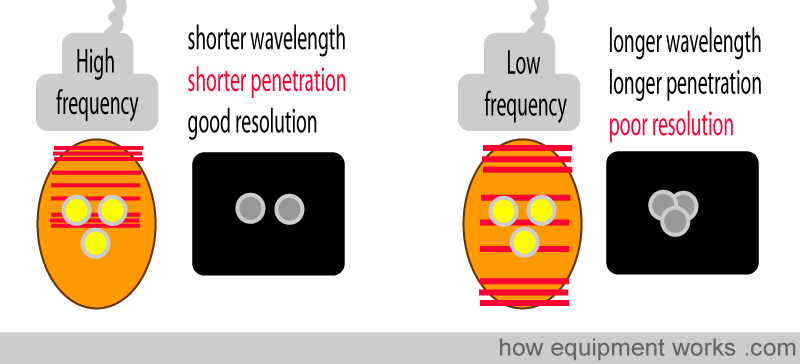
So when choosing the optimum frequency, we have to choose somewhere between the needs of a good depth of penetration and a good resolution.
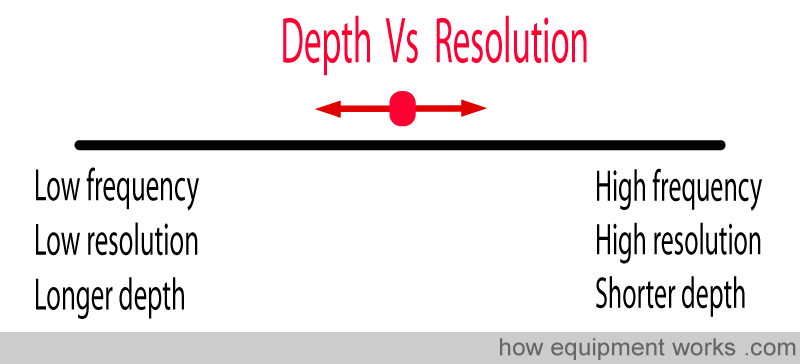
If the frequency is too high, the depth of penetration of the ultrasound will be inadequate and you may not see the structure you want.
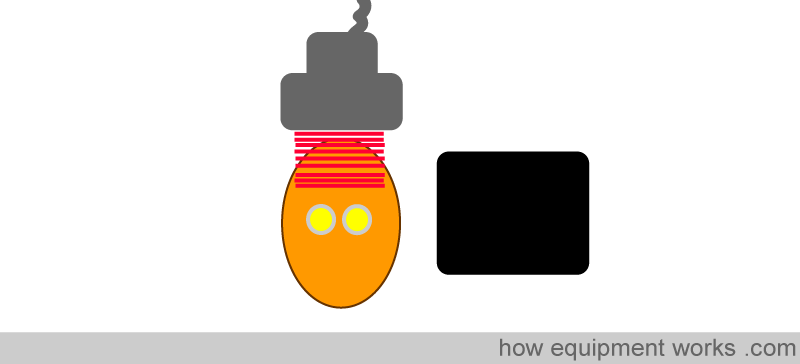
If the frequency is too low the ultrasound waves will penetrate deeper than necessary. The unnecessarily low frequency will give you a low resolution image that will be blurred.
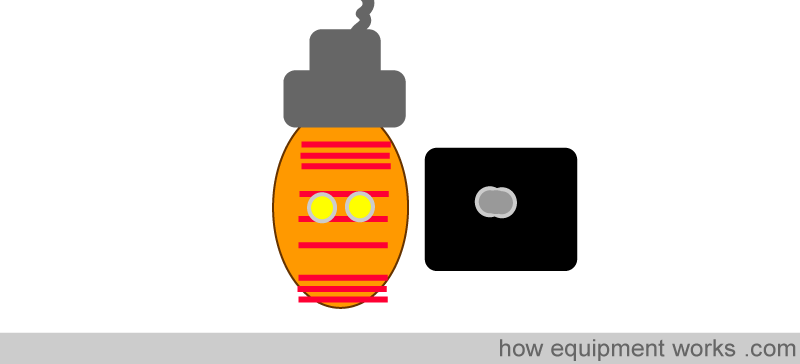
The optimum frequency is one that will give you just the adequate amount of depth necessary to see the structures of interest. You don’t allow unnecessary depth which would have unnecessarily lowered the frequency.
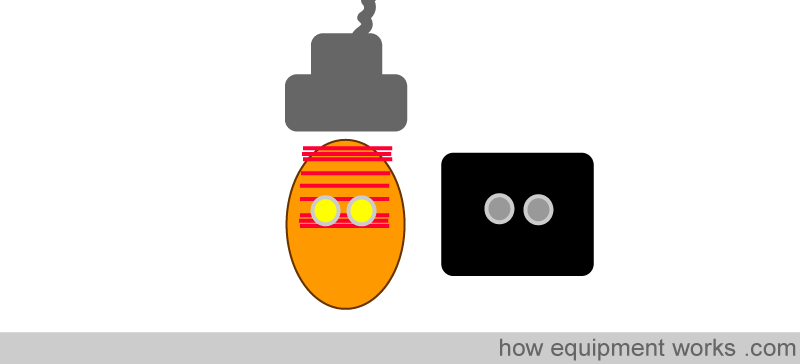
To summarise:
Correct depth = reasonable high frequency = reasonably short wave length = reasonably good resolution.
Too much depth = low frequency = long wavelength = poor resolution
Too little depth = Wont see structures of interest !
Doppler Effect
Before proceeding to explain the Doppler effect, let us quickly revise what frequency means. Frequency is the number of oscillations of the sound wave that occurs per one second. In the example below, the high frequency has four oscillations per second and the low frequency has two oscillations per second.
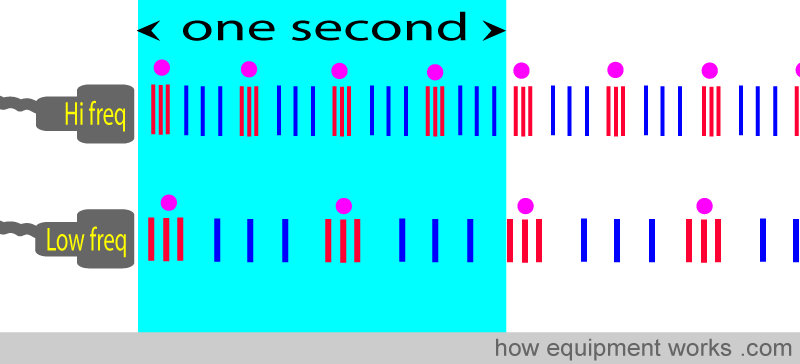
Now let me try and explain the Doppler effect. As you will recall, the ultrasound machine measures the distance to things by transmitting ultrasound waves from the probe and seeing how long the wave takes to return back to the probe.
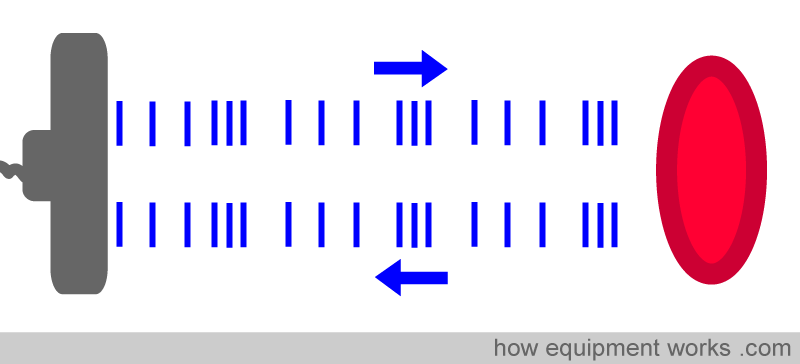
The transmitted wave has a certain frequency. The wave that returns to the probe also has a certain frequency. When the wave is bounced back from a stationary object such as a nerve, both the transmitted and the returned waves have the same frequency.
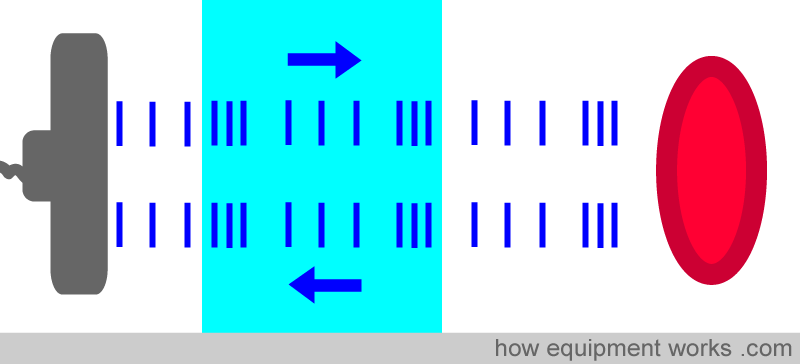
However, if we repeat the same thing with an object moving towards the probe, something interesting happens. Imagine that the red disc below is an red blood cell moving towards the probe ( I know it is a rather large red blood cell !).
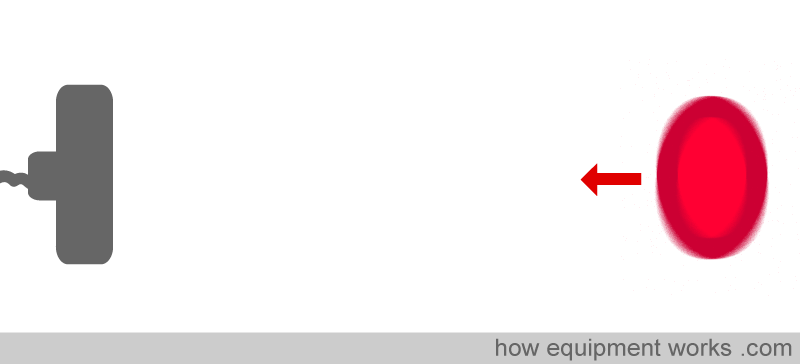
Again we send out an ultrasound wave.
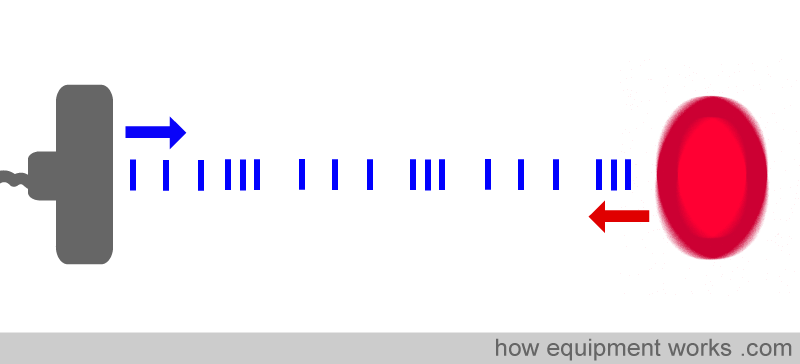
This ultrasound wave reaches the moving red blood cell and bounces back. However, this time if you measured the frequency of the returned wave, it will not be the same as the frequency of the transmitted wave. The wave that bounces off an object moving towards the probe will have a higher frequency than the frequency of the wave transmitted from the probe. This is becuase the moving object “squashes” the waves as it moves towards the probe (see diagram below) . This is an example of the doppler effect. When a wave is sent to an object that is moving towards the transmitting probe, the doppler effect makes the frequency of the returning wave to be higher than the frequency of the wave sent out. The faster the object moves towards the transmitting probe, the higher will be the difference.
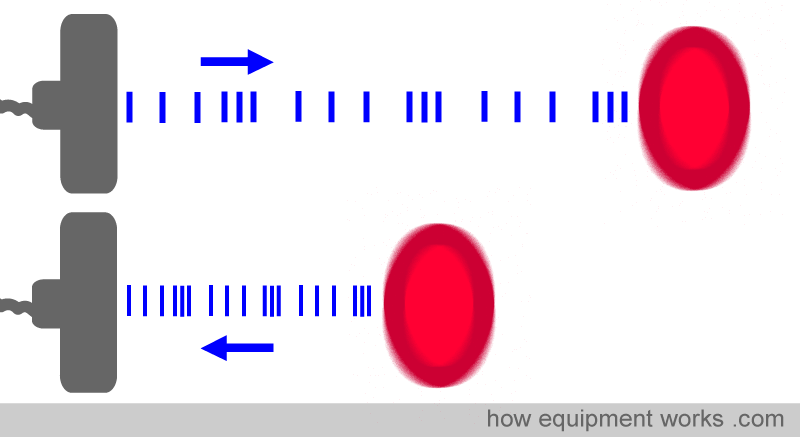
Ultrasound imaging devices can use the doppler effect to help us in many ways, including helping us to identify blood vessels.When you scan structures without using the doppler effect, the machine simply sees how long the waves take to return back to the probe and constructs an image.
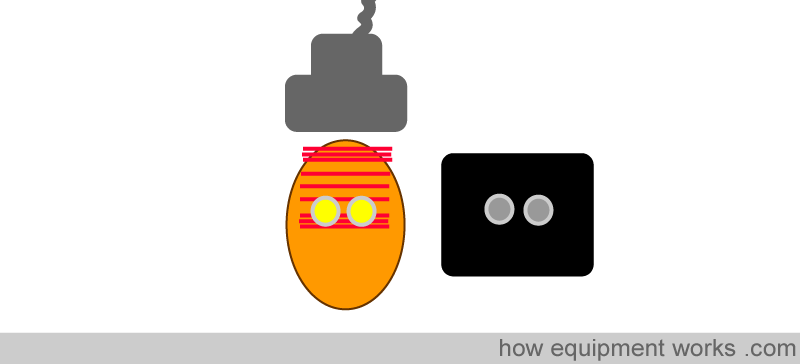
However, this image does not clearly show which of the circles is a blood vessel and which is a nerve. Fortunately, blood vessels have one big difference from nerves. Blood vessels are full of rapidly moving red blood cells. When using an ultrasound machine with the ability to look for the doppler effect, the machine constructs an image in the usual way by seeing how long waves take to return back to the probe. But in addition, it also analyses the frequency of the returned waves. Whenever the returned wave has a frequency different to the frequency of the transmitted wave, the machine knows that the place where those waves bounced back from have moving objects. To help you to see these areas of moving cells, the ultrasound machine “adds colour” to areas showing the “doppler effect”. In the image below, this helps you to differentiate nerve from blood vessels. The nerve has no moving cells, so there is no doppler effect and therefore no colour is added by the machine. The blood vessel has rapidly moving cells which cause a doppler effect, and where this occurs, the machine adds the colour red to help you identify it. This makes it easy for you to identify the blood vessel.
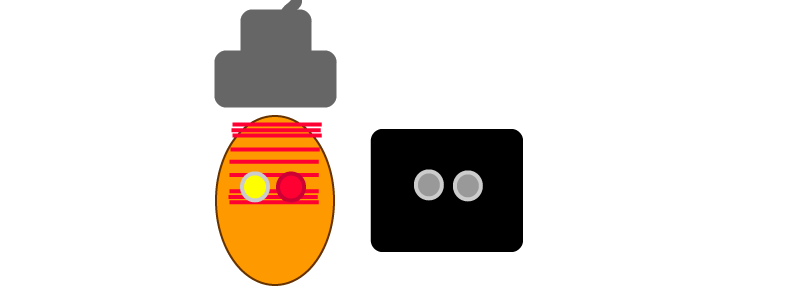
Let me try and explain how the doppler effect happens. When an ultrasound wave meets something moving towards it, the wave gets ‘compressed’ by the object.
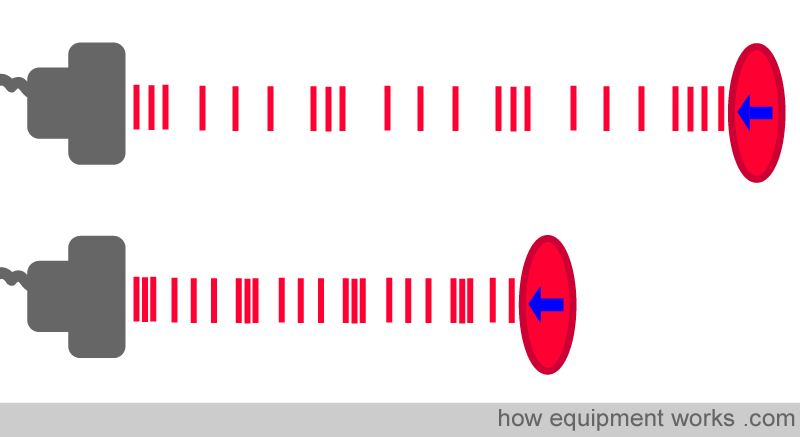
The compression of the wave into a smaller length means that the oscillations of high and low pressure areas of the wave become more concentrated. As the wave gets compressed, it has more oscillations (high pressure / low pressure areas) per second than before ( i.e. the frequency has increased). This explains how the frequency of waves reflected from objects moving towards the probe have an higher frequency than the frequency of the wave sent out.
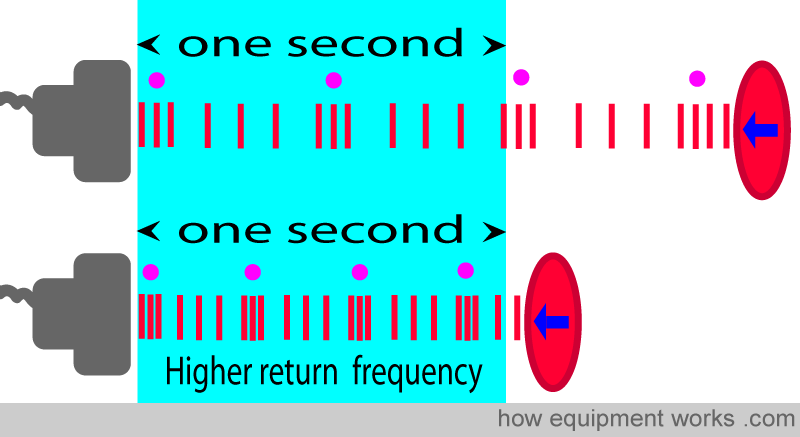
The Doppler effect also occurs for objects moving AWAY from the transmitting probe. Again there is a difference between the frequency of the transmitted waves and the frequency of the returning waves. However this time , the returning waves have a LOWER frequency than the frequency of the waves transmitted. The faster the object moves away, the greater will be the frequency difference. The reason for this decrease in frequency is the opposite of the explanation given before. In this case, the object moving away ‘stretches’ the wave. The stretching reduces the number of oscillations per one second.
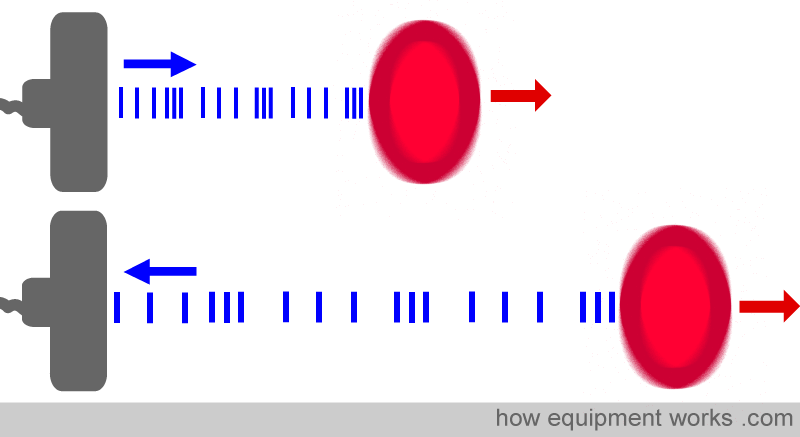
To summarise, the doppler effect causes the frequency of waves reflected from a moving object to be different from the frequency of the wave sent out of the probe.
If the object is moving towards the probe, the reflected frequency is increased.
If the object is moving away, the reflected frequency is decreased.
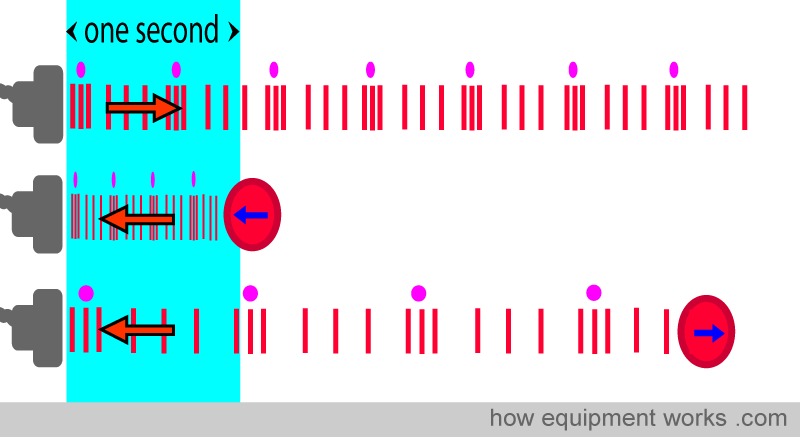
Ultrasound machines tell us doppler effect information using colour. It uses different colours to show the direct and speed of flow. This helps you to identify vessels.
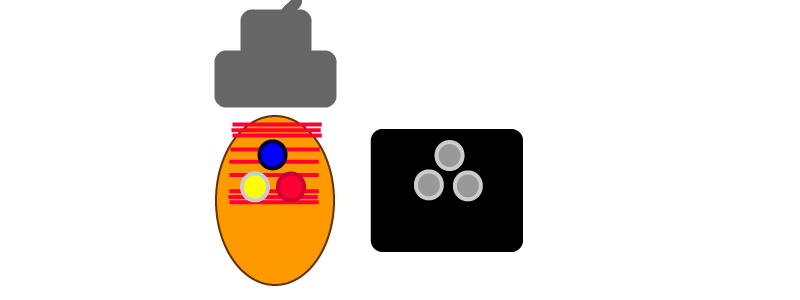
We have now reached the end of our discussion on the basics of ultrasound physics. I hope it has given you a good introduction to the subject and will help you when you read further on this topic.
Bye and hope to see you soon in a another section of the website.

Hello! My name is Pras and I am the author of this website that you are now reading. I have made this website completely free to access so that people from all over the world can benefit from it.
If you can afford it, I would be very grateful if you would consider making a single donation of one dollar (or the equivalent in your currency) to help cover the expenses needed to run this website (e.g. for special software and computers). For this website to survive, donations are desperately needed. Sadly, without donations, this website may have to be closed down.
Unfortunately, perhaps because many people think that someone else will donate, this website gets only very few donations. If you are able to, please consider making a single donation equivalent to one dollar. With support from people like you, I am sure that this educational website will continue to survive and grow.
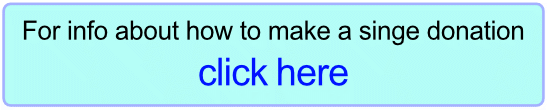
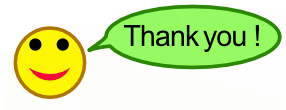